


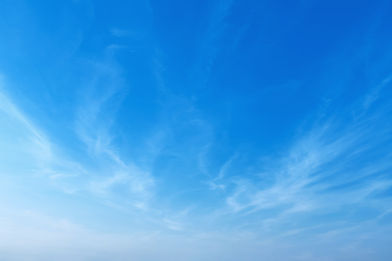
Engineered Cell and Gene Therapy Platform
Human gene therapy is a medical treatment of a genetic disorder by altering genetic material in a patient to correct the underlying genetic problem. Gene therapy products are being investigated to treat diseases including cancer, genetic diseases, and infectious diseases. In the past three decades, the field of gene therapy has been recognized with many hurdles and setbacks. Gene therapy must overcome complex tissue and cellular barriers to introduce genetic modifications with sustained clinical benefit. Contemporary viral vector-based gene therapy has relied on adenoviruses, adeno-associated viruses, and lentiviruses for the delivery of the therapeutic genes. Several drugs based on viral vectors including one oncolytic virus have gained regulatory approval by the FDA. However, gene therapy is costly and still presents significant technical challenges, including the complexity of manufacturing the therapeutic agents and potential safety risks of insertional mutagenesis. In addition, the generation of antibodies against virus-delivered therapies can result in a robust immune response, which limits their ability to any subsequent dose. Strikingly, gene therapies combined with nanoscale delivery systems have broadened the therapeutic and biomedical applications of these regimens.
To achieve safe, potent, and durable therapeutic benefits, cell-based immunotherapies are designed to improve the endogenous cytotoxicity of immune cells to attack cancer. Many types of cellular therapy for cancer are being explored, including chimeric antigen receptor (CAR) T cells, other genetically modified T cells, tumor-infiltrating lymphocytes (TIL), natural-killer (NK) cells, and vaccines. Despite notable recent successes against certain hematological malignancies and some autoimmune diseases, autologous cell therapies continue to face numerous challenges that limit their patient accessibility and commercial spaces. The field of genetic engineering, particularly advancements in gene-editing technologies and synthetic biology, is poised to enhance patient care by introducing new therapeutic modalities such as allogeneic cell therapy. Ongoing research at Cohnps Co. is building industry-leading capabilities by investigating a novel proprietary platform, particularly within the realm of non-viral intervention strategies, for future drug discovery and disease management. These will be first-in-class therapeutics designed specifically to eliminate malignant cells towards cure. A cure for cancer will no longer be our culture’s threadbare metaphor for a miracle, but rather within our reach.
Engineered Alternative Nanobody Platform
Traditionally, a monoclonal antibody (mAb) against a specific antigen is produced by B cells driven by advances in hybridoma technology, and the development of mAbs has played a prominent role in modern clinical therapeutics, diagnostics, and biomedical research. Though antibody engineering can be used to strengthen the efficacy of antibodies, reduce their immunogenicity, and prolong mAb half-life, antibody engineering technologies face increasing demands for speed, reliability, and scale. More recently, the functionally engineered antibody domains have been leveraged to generate a fragment antigen binding (Fab), F(ab')2 fragments, single-chain variable (scFv) fragments (scFabs), or single-domain antibody fragments (VHHs or nanobodies) derived from the heavy chain-only antibody of camels to enhance their performance for diverse applications. In addition, Abdurin is derived from the second heavy-chain constant domain (CH2) of human immunoglobulin G (IgG) as a stable monomer in the absence of glycosylation. The Fab moiety of the IgG is the most used choice for assembly of multifunctional antibodies, while scFV and VHH are frequently applied to bispecific T-cell engagers (BiTEs) and CAR T-cell based therapies. The single-domain antibody (VHH) takes great advantages of stability and penetrability, which are essential to the development of several antibody drugs or diagnostic tools as promising targeted imaging probes. The VHH has different binding properties than conventional antibodies, and construction of a synthetic, humanized VHH library can reproduce the structural properties of camel VHHs for treatment utilities. Nowadays, camelids (e.g., dromedaries, camels, llamas, and alpacas) and even sharks can be immunized as repertoire sources to develop single-domain antibodies against targets of interest. To date, three Fab drugs (abciximab, idarucizumab, and ranibizumab) and two VHH drugs (caplacizumab and ciltacabtagene autoleucel) have been approved by the FDA, but no Fab or F(ab’)2 has been approved for cancer treatment. In addition, targeted delivery of covalently linked therapeutic payloads with monoclonal antibodies or antibody-drug conjugates (ADCs) is an important and widely accepted platform of modern drug development. In the past decade, unprecedented advances in immuno-oncology have been made by unleashing the natural power of a patient’s immune system to fight cancer. Researchers continue to harness the power of molecularly targeted therapy, ADCs and bispecific antibodies (BsAbs), and to advance cancer immunotherapy of checkpoint-blockade antibodies, BiTEs, and immunocytokines alongside chemotherapy, radiation, and surgery.
When antibodies are generated and produced without animal immunization, they are regarded as synthetic antibodies. Robust and advent of non-IgG protein scaffolds as antibody alternatives for novel binding functions emerged two decades ago. Engineered protein scaffolds are developed from human protein fragments with specific affinity of target binding properties, exceptional stability, and various physicochemical properties of biomedical relevance. With combinatorial protein engineering by varying amino acid sequences at exposed surface sites with select regions of the scaffold binding proteins, highly diversified random libraries of different variants can be created with low-cost production and rapidly screened for variants with high specificity and avid affinity against different targets. Some examples of protein scaffolds include ADAPT, Adnectin (Monobody), Affibody, Affilin, Affimer, Anticalin, Avimer, Bicycles, Centyrin, DARPin, Fynomer, Knottin, and Kunitz, and to date, more than 20 types of protein scaffolds have been developed. Engineered protein scaffolds can be mass-produced via the prokaryotic expression systems that are more cost-effective. Most importantly, the small size (only 12-15 kDa molecular weight) leads to greater and more rapid tissue penetration, reduced risk of immune system activation, and the ability to better recognize epitopes on protein targets. However, the small size of many engineered protein scaffolds as well as the single-domain antibodies and nanobodies demonstrated their very short plasma half-life due to rapid renal clearance. Several approaches are used to increase the circulating half-life of the various scaffolds, including albumin association or a fragment crystallizable (Fc) fusion and the addition of half-life extension peptides or polyethylene glycol (PEG) (Pegylation). Nevertheless, the size advantages are lost because such modifications increase the molecule’s size to avoid renal clearance. Despite some protein scaffolds showing promising performance in different fields, few can realize successful clinical translation for cancer therapy and imaging applications. Cohnps Co. is seeking to construct a transformative proprietary platform by creating superior nanobody mimetics with many favorable properties to yield highly desirable diagnostic tools and more effective treatment agents against across a broad range of diseases. We will maximize the power of machine learning and artificial intelligence (AI) by generating high-throughput datasets of complex alternative nanobodies to deliver unique insights into precision engineering for redefining the antibody drug development paradigm that drives cancer cure.
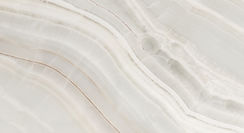